In 2018, roughly a year before the World Health Organization declared Covid-19 a global health emergency, a conference in Edinburgh imagined a very different type of medical crisis: during a three-year mission to Mars, there are growing cases of an unknown vision problem among the 40 people temporarily living on the planet. As more people start experiencing blurry vision, some can no longer perform their mission duties, and many begin to panic. The conference—called Clinical Trials for Interplanetary Missions—brought together trialists, clinicians, and psychologists to brainstorm how clinical research could work in this deep space mission.
Though this scenario may seem like far-fetched science fiction, experts say humans will likely set foot on Mars within the next two decades. Meanwhile, analysts predict over $1 trillion of the global economy will move into space by 2040, fueled in part by the expected takeoff of commercial spaceflight. Yet our understanding of how the human body responds to medicine in space is still extremely limited.
As engineers build the technology to transport more humans to space and eventually Mars, scientists need to develop the pharmaceutical tools and medical understanding to support these missions in parallel, says Li Shean Toh, PhD, a pharmacist at the University of Nottingham researching medicine in space. Doing so, she adds, will require intense collaboration from scientists, commercial space companies, and space agencies across the planet. “Many people say this level of cooperation is impossible,” she says. “But people also said landing on the moon was impossible.”
Dosing drugs in outer space
During our evolution, humans were shaped by Earth’s gravity and atmosphere, explains Dr. Thais Russomano, a professor of space physiology and CEO of the think tank InnovaSpace. “Once humans enter space, every single cell and every single cellular process change,” she says. “This could really alter the way we process medicines.”
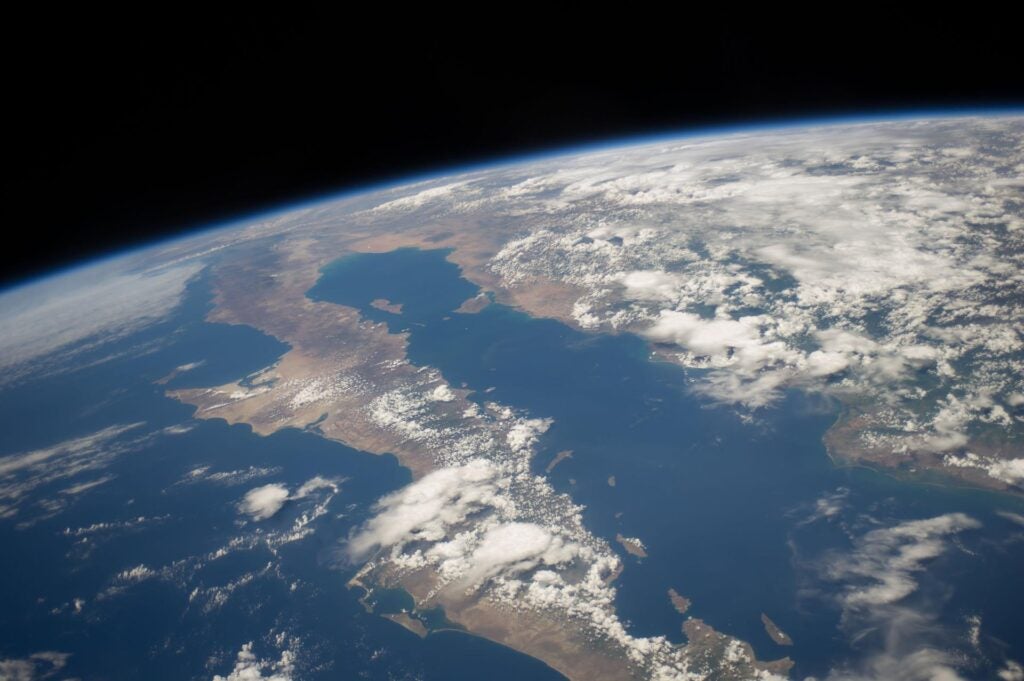
When humans experience microgravity in space, their body fluids redistribute to the upper parts of the anatomy, explains Dr. Emmanuel Urquieta, a professor at Baylor College of Medicine’s Translational Research Institute for Space Health. The cardiovascular system then compensates by increasing red blood cell levels, which in turn limits fluid production and increases urination. Microgravity also causes space adaption syndrome—a type of nauseating motion sickness—that can significantly alter the gastrointestinal system, he adds.
How well do you really know your competitors?
Access the most comprehensive Company Profiles on the market, powered by GlobalData. Save hours of research. Gain competitive edge.
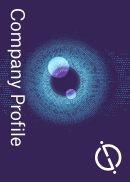
Thank you!
Your download email will arrive shortly
Not ready to buy yet? Download a free sample
We are confident about the unique quality of our Company Profiles. However, we want you to make the most beneficial decision for your business, so we offer a free sample that you can download by submitting the below form
By GlobalDataBecause blood flow and digestion rate alter how the body processes drugs, normal doses on Earth do not necessarily translate to the required doses in space, notes Dr. Christoph Seubert, an anesthesiologist at the University of Florida who has worked on NASA-funded research. So far, there have been no serious medical emergencies in space. But most astronauts are only in space for short periods of time and take low-risk, over-the-counter drugs, where unintentional overdoses are unlikely to cause serious issues. However, for astronauts with serious medical emergencies on a deep space mission—or for non-astronauts with underlying health conditions on commercial spaceflights—exact dosing becomes critical, Seubert says.
Traveling beyond the moon
When humans travel to deep space—defined as anywhere beyond the dark side of the moon—the biological changes will likely be even greater. The most important concern is long-term exposure to cosmic radiation from the sun, which could damage cellular function and cause cancer, Urquieta explains.
The Earth’s magnetic field blocks most cosmic radiation, and even the International Space Station (ISS) has some radiation protection due to its proximity to Earth. But on a Mars mission outside of low-Earth orbit, there is almost no protection against radioactive particles. “Toxic space radiation is very difficult to shield against because it’s pretty much traveling at the speed of light,” Urquieta says.
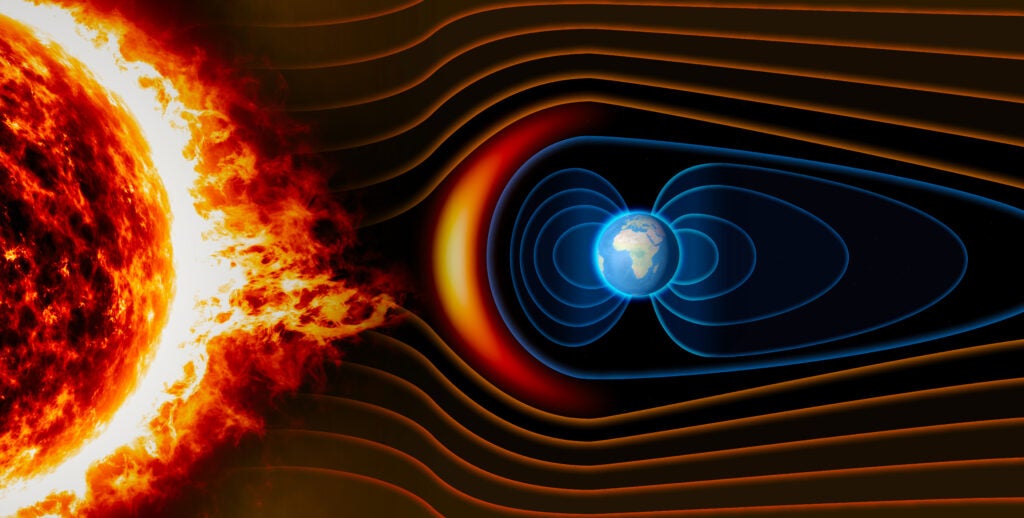
The isolating environment of long-term space travel could also cause serious psychological stress, further altering how medicine affects the body, Urquieta adds. Irregular light patterns and motion sickness can disrupt astronauts’ circadian rhythms, leading to poor sleep quality that compounds the mental health effects of confinement. More research into how terrestrial drugs for mental health conditions, such as antidepressants and sleep aids, affect the body in space is crucial preparation for a deep space mission, he says. “In a 900-day mission to Mars, there will be four astronauts living on a relatively small vehicle,” he notes. “The behavioral health implications are very important to understand.”
To make matters worse, communication between Earth and a Mars-bound spacecraft could take up to 40 minutes, removing the possibility of real-time conversations, Urquieta says. There will also be up to two weeks where communication to Earth is impossible—while the sun is directly between Earth’s orbit and the spacecraft. In many cases, he notes, artificial intelligence will have to substitute for human doctors.
Designing extraterrestrial trials
As an important step to understanding how drugs work in space, humans should conduct classical pharmacokinetic studies in low-Earth orbit, explains Virginia Wotring, PhD, a space pharmacologist at the Baylor College of Medicine who has worked with NASA and the European Space Agency (ESA). Astronauts already take common medications aboard the ISS, making the floating laboratory an ideal starting point for pharmaceutical research, she says.
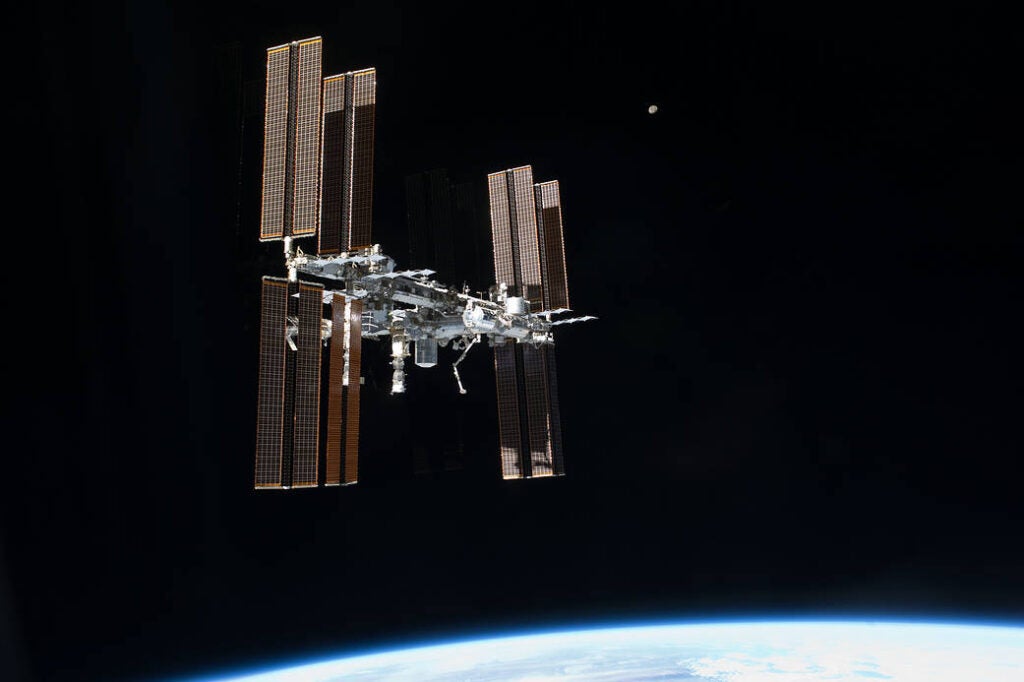
However, even basic tests of drug activity require significant adaptations for space, Toh notes. Intravenous blood sampling—the preferred biomarker of drug activity on Earth—is far riskier and more invasive in the space environment. As a result, past astronaut studies have relied on saliva samples, which paint a much weaker picture of a drug’s activity than a blood sample. Ongoing research into more sensitive, less invasive biomarker monitoring alternatives—like fingerprint-based tests—could pave the way for more effective pharmacology studies, she says.
To conduct even more complex clinical studies in space, trialists will need to think outside the bounds of traditional designs. Luckily, Seubert says, we already have some experience on Earth: “We do drug trials for rare diseases and extreme terrestrial environments, so the methodologies for space are already there.”
One option is a personalized clinical trial, which clinicians already use for ultra-rare diseases, Seubert explains. In an N=1 trial design, a single patient takes a medication, using prespecified outcome measures and biomarkers to determine a drug’s safety and efficacy. Another option is a Phase 0 trial, which entails micro-dosing a medication over a short time period, explains Dr. Tal Burt, a drug development consultant. Just one week of micro-dosing can improve understanding of how the body processes a drug in space—without the safety risks and lengthiness of a traditional full-scale trial, he explains.
Manufacturing drugs for deep-space
Once scientists discover how drugs work in space, they will likely have to figure out how to make them in a small spacecraft. On Earth, many pharmaceuticals expire within a year and six months. A Mars mission, however, could take twice as long, and spacecraft will not have the storage capacity to carry several years’ worth of emergency drugs, Urquieta says.
To solve this problem, scientists are exploring ways to artificially synthesize pharmaceutical compounds, either through genetically modified plants or bacteria. At UC Davis, researchers have already genetically modified lettuce to synthesize a drug that protects against bone density loss. This builds upon previous research where astronauts successfully harvested and ate red romaine lettuce aboard the ISS. Eventually, Urquieta says, astronauts could travel to space with a gene bank, injecting specific genes into lettuce seeds when they need to synthetically grow a new pharmaceutical.
Meanwhile, other researchers are exploring new medicines specifically tailored to the unique challenges humans face in deep space. Scientists have already sequenced the genome of tardigrades—micro-animals that can withstand ionizing radiation hundreds of times greater than the lethal dose to humans—to learn how humans could build more radiation resistance. In one experiment, researchers found stress-related tardigrade genes can be transferred to human cell cultures, setting the stage for potential applications in both spaceflight and cancer. In another particularly shocking experiment, the ESA sent 3,000 tardigrades into the vacuum of space for 12 days outside of a spacecraft—and over two-thirds survived.
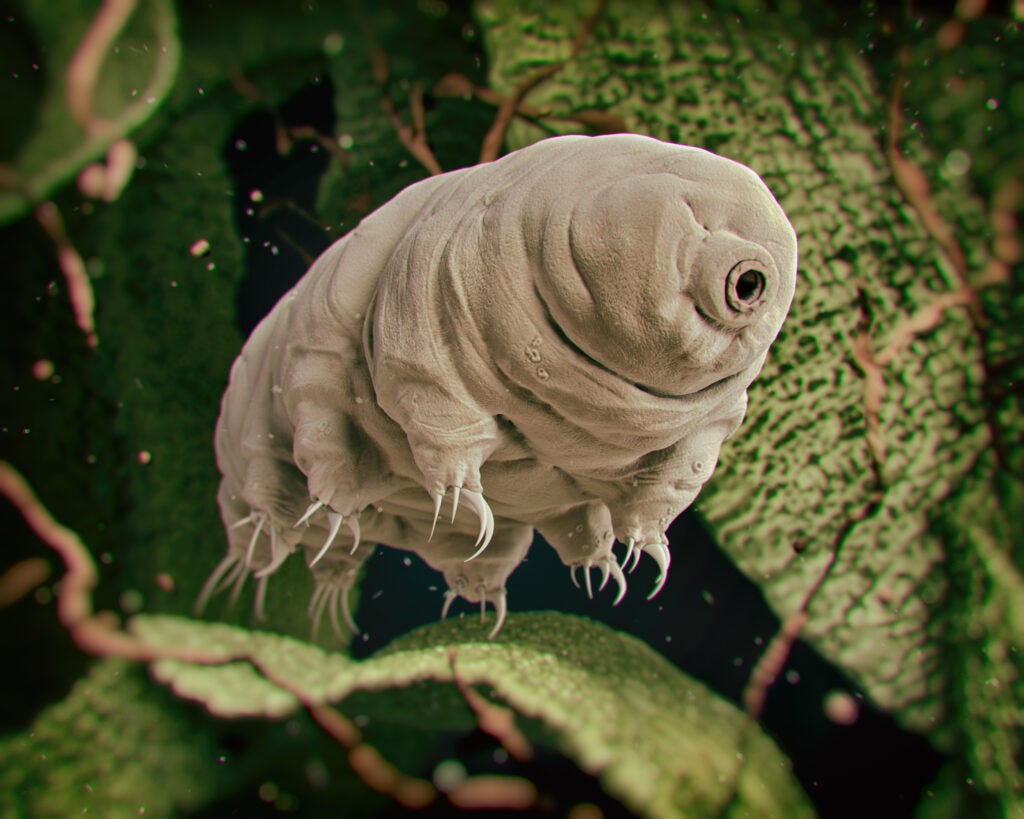
A new space race?
After the Cold War rivalry between the US and USSR fueled the beginnings of space exploration in the 1960s, a new era of international space cooperation ensued. The ISS—a collaboration between the US, Europe, Russia, and Canada—has been a revolving door of international scientists since it launched in 1998.
The future of space exploration, however, could become fragmented once again, as analysts expect old geopolitical rivalries to regain their footing. As the ISS slowly succumbs to the wear and tear of space, NASA plans to decommission the symbol of international cooperation by 2031. Meanwhile, several private companies—including Elon Musk’s SpaceX and Jeff Bezos’s Blue Origin—are beginning to replace government agencies as the most dominant players in space, adding a new level of complexity to global collaboration efforts.
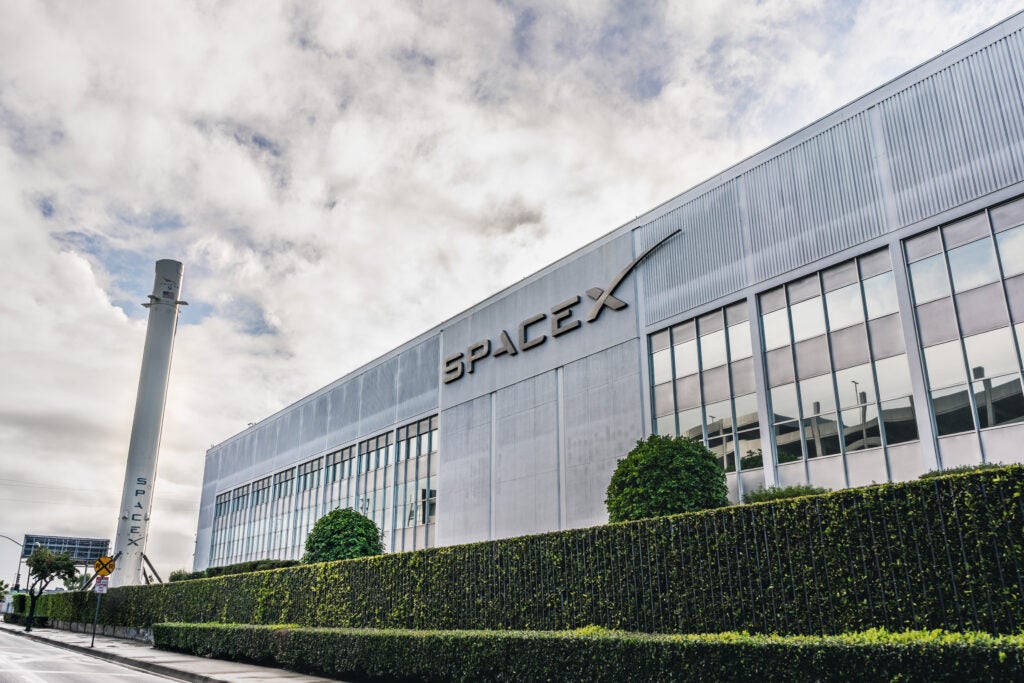
As scientists work to build the pharmaceutical tools for the future of space travel, data sharing and patient privacy are becoming key concerns, Toh says. Each major country has its own rules governing patient privacy, many of which require patient-level data to be de-identified so it cannot be traced back to an individual person. Given the small numbers of people in space, it becomes very difficult to protect privacy while transparently sharing data to advance knowledge, she says.
“We need to build a true global consensus and a global protocol for managing medical data in space,” Toh says. “Right now, the data is still very secret because there’s so much pressure on the industry to succeed.”
Coming back to Earth
Whenever humans invest in space research, unexpected new technologies on Earth tend to follow. LASIK surgery, artificial limbs, cochlear implants, and infrared ear thermometers, among other healthcare innovations, all have their origins in NASA-funded research. The same could be true for the drug development and manufacturing industries, as the next era of research in aerospace medicine is sure to prompt unanticipated discoveries.
At the Clinical Trials for Interplanetary Missions conference in Edinburgh, participants quickly realized that top-down models for clinical trial design on Earth were unfeasible for a crisis on Mars, explains Mona Nasser, PhD, who organized the event. Instead of rigid trial protocols from outside companies, they imagined adaptive study designs where patients were empowered to make important healthcare decisions about the treatment candidates they receive. Many of these same strategies, the participants concluded, could also improve clinical trials back home.
“We quickly realized that we have to change the way we do medical care to put patients first,” Nasser says. “If we want a better future in healthcare, we have to be more inclusive, whether it’s on Earth or on Mars.”